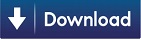

Group14 began construction of a 20-gigawatt plant in Moses Lake, Wash., in April. All three plan to have domestic gigawatt-scale factories up and running in the next few years. Department of Energy and, for Group14, another $214 million in private investment. In late 2022, Group14, Sila, and Amprius Technologies in Fremont, Calif., raised nearly half a billion dollars to commercialize their anode materials, with US $250 million from the U.S. Group14 Technologies, in Woodinville, Wash., should have its silicon battery setup in a Porsche EV by next year. Alameda, Calif.–based Sila Nanotechnologies’ silicon anode, which has powered the Whoop fitness tracker since 2021, will be in the Mercedes G-Class SUV by 2026. General Motors and OneD Battery Sciences in Palo Alto, Calif., are putting OneD’s silicon nanotechnology into GM’s Ultium battery cells. Some carmakers and silicon anode startups have teamed up to produce longer-range, lower-cost EVs that could be on the road by mid-decade. “Silicon has transformed the way we store information, and now it’s transforming the way we store energy.” –Rick Costantino, Group14 Now, however, after some 15 years of incremental improvements and dashed hopes, silicon’s time as a mainstay material in batteries has finally arrived. But experiments with that element have been plagued by technical challenges-including volume expansion of the anode when loaded with lithium ions and the resulting material fracture that can happen when an anode expands and contracts. In fact, silicon’s first documented use as a lithium battery anode even predates that of graphite- by seven years. Silicon has long held out promise as a medium for anodes, because it can hold 10 times as many lithium ions by weight as graphite. Lithium-ion batteries’ graphite anodes, by contrast, have largely stayed the same.

And yet even for the technology’s vast advancements-a staggering thirtyfold drop in price between 19, for instance-the biggest improvements have taken place mostly on the lithium-metal-oxide cathode side.
CATHODE CHARGE PORTABLE
This indicates that electrolytic solution plays a significant role in the surface reconstruction phenomenon.Since lithium-ion batteries’ commercial debut three decades ago, this portable and high-density (and Nobel Prize–winning) energy storage technology has revolutionized the fields of consumer electronics, electric vehicles, and large-scale energy storage. Less surface reconstruction to rock salt is observed for chemically delithiated samples compared with electrochemically delithiated ones. Both chemical and electrochemical delithiation methods lead to state-of-charge non-uniformity in the bulk of layered transition metal oxide cathode particles. The present study not only strongly implies that the electrolytic solution is an active participant in the reactions that lead to the surface reconstruction to a rock salt structure observed in layered oxide cathode materials, but also develops a chemical basis for understanding and improving charge homogeneity across the length scale of secondary particles. The two types of materials show similar bulk lithium distributions but distinctly different surface chemistries. In our work, bulk and surface-sensitive characterization techniques were used to investigate the differences between chemically and electrochemically delithiated layered cathode materials. The surface chemistry and bulk lithium distribution of chemically delithiated samples may not accurately represent the electrochemically charged materials in a real battery.
CATHODE CHARGE FREE
Chemical delithiation is extensively used in preparation of charged cathodes free of inactive components for fundamental battery studies. However, the gradient oxidation states of transition metals on the surface, which is partly responsible for the electrode degradation mechanism known as surface reconstruction, is much less apparent in chemically delithiated samples. Therefore, the chemically delithiated samples are similar to the electrochemically charged samples in terms of mesoscale charge heterogeneity in large polycrystalline particle ensembles. We observed considerable and similar non-uniformities in terms of Ni oxidation states (and, by proxy, lithium distributions) for all the samples in the bulk. Nanoscale full-field (FF) transmission X-ray microscopy (TXM) and ensemble-averaged soft X-ray absorption spectroscopy (soft XAS) were used to investigate state-of-charge (SOC) heterogeneities in electrochemically charged or discharged and chemically oxidized samples of LiNi0.6Mn0.2Co0.2O2 cathode materials.
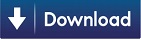